Some geochemical
features of carbonatite baddeleyite from Kovdor Massif (Kola Peninsula)
Rodionov N.V.*,
Belyatsky B.V **, Antonov A.V.*, Simakin S.G.***,
Kapitonov I.N.*, Sergeev S.A.*
*CIR
VSEGEI, S.Petersburg, Russia,
**VNIIOkeangeologia,
S.Petersburg, Russia,
***YaB
PhThIAN, Yaroslavl, Russia
bbelyatsky@mail.ru
Recently baddeleyite (ZrO2)
has been introduced into practice of U-Pb dating of basic, ultrabasic
and alkaline intrusive rocks [Heaman, 2009; Rodionov et al., 2012]. It
is known that its crystallization from magmatic melts is determined by
low SiO2 activity at rather high Zr concentrations and at the
same time its occurrence together with zircon in polyphase alkaline-ultrabasic
intrusions evidence to more complicated and variable genesis of this
mineral. In many cases composition of trace elements may be used as
genetic indicator for the origin not only of rock-forming minerals but
accessory minerals as well. Zircon trace elements composition is widely
used in geochemistry [Hoskin, Ireland, 2000],
but as for baddeleyite, its geochemical characteristics which reflect
the peculiarities of crystallization and origin are still insufficiently
known [Klemme, Meyer, 2003]. For example, the published data on
composition of rare-earth elements (obtained both by in-situ and
bulk sample analysis of this mineral) are debatable and in many cases
contradictory. Results of in-situ analysis of separate
baddeleyite grains prove the main geochemical properties of this
mineral, but even for baddeleyite from Phalaborwa massif which is often
used as geochemical standard, the results from different laboratories
vary significantly [Rodionov et al., 2012]. In particular, the shape and
value of Ce anomaly differs more than in order, in many REE patterns the
Eu anomaly is absent and the range of REE fractionation differs from the
results obtained by other analytical methods. At the same time, many
scientists consider baddeleyite from the Kovdor massif as a potential
standard for geochronological studies. This has stimulated the present
study of in-situ geochemical composition of representative
baddeleyite grains from the Kovdor massif.
It is well known [Rimskaya-Korsakova,
Dinaburg, 1964; Kirnarsky, 1979; Kopylova et al., 1980] that baddeleyite
in the rocks of alkaline-ultrabasic Kovdor complex is ubiquitous mineral
and it is formed at all stages of rocks formation from olivinites to ore
phoscorites and postmagmatic syenites [Dudkin, Kirnarsky, 1994; Krasnova,
Kopylova, 1988]. Morphology of baddeleyite varies greatly – from large,
several centimeters, black crystals to light colored yellow
semitransparent sub-millimeter grains [Kirnarsky, 1979]. We have studied
in-situ compositions of more than 50 different in morphology
baddeleyite grains from the carbonatite and phoscorite samples from the
Kovdor massif and 20 baddeleyite grains from the Phalaborwa carbonatite
massif (South Africa) for comparison and test of the approach
applicability (ion microprobe CAMECA IMS-4F and laser-ablation with
ISP-MS Element2). Our results of baddeleyite REE composition are
presented in the summary diagrams on Fig.1 and the most important are
formulated below.
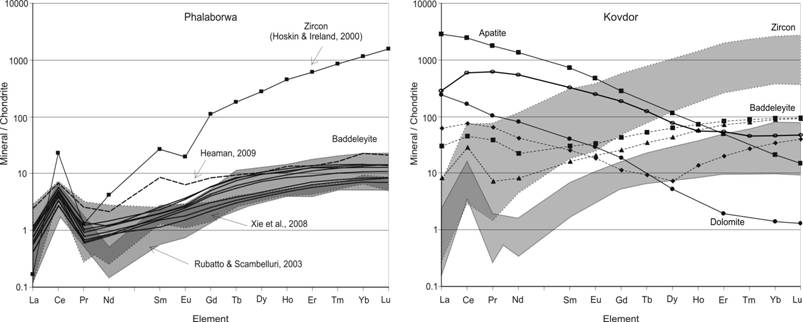
Fig.1. REE patterns for
baddeleyite from carbonatite complexes: (а)
Phalaborwa (S.Africa), for comparison data
from Heaman, 2009; Hoskin, Ireland, 2000; Rubatto, Scambelluri, 2003;
Xie et al., 2008 are shown; and (b) Kovdor (Kola Peninsula), open
circles – ISP-MS data for 100 mg baddeleyite sample.
As above mentioned, the
published data on baddeleyite trace element composition are scarce even
for the “Phalaborwa” U-Pb standard [Heaman, 2009; Rodionov et al.,
2012]. According to a few studies on the “Phalaborwa” standard (∑REE =
10.6–22.4 ppm) baddeleyite REE composition displays significantly lower
HREE than those typical for magmatic zircon and other baddeleyites and
less fractionated: (Lu/La)n = 8.4–20.1, (Lu/Gd)n=
2.5–4.2, (Sm/La)n= 2.4–3.5 [Reischmann et al., 1995; Rubatto,
Scambelluri, 2003; Xie et al., 2008] (Fig. 1a). This agrees well with
experimental data on the composition of baddeleyite crystallized from
carbonatitic melt [Klemme, Meyer, 2003]. Our trace element data for the
Phalaborwa baddeleyite are quite similar to the earlier published
in-situ analyses and reflect relatively limited variation of their
compositions and acceptable reproducibility of the chosen microanalysis
methods (Fig. 1a).
But the LREE patterns for
Phalaborwa baddeleyite are considerably different from the data of
Reischmann et al. (1995), obtained with a combination of spark source
and thermal ionization mass spectrometry techniques, with an unusual
U-shaped REE pattern without a Eu-anomaly. It is supposed that analyses
are compromised by inclusions and impurities in the studied baddeleyite
separates [Reischmann et al., 1995]. But the chondrite-normalized REE
patterns for studied Kovdor baddeleyite are more diverse with some of
them resemble the published U-shaped distribution for Phalaborwa
baddeleyite (Fig.1b). In accordance with abovementioned suggestions we
attribute these deviated REE distributions to the influence of the
micro-inclusions (mostly apatite and carbonates), the presence of which
was confirmed by SEM-EDX analyses for some baddeleyite grains. REE
patterns for large apatite and dolomite inclusions located inside
baddeleyite crystals confirm such interpretation, since their REE
composition patterns exhibit strong LREE enrichment, similar to those
reported earlier by Kempe and Götze (2002) and Arzamastsev et al. (2009)
for these minerals from the Kovdor and Phalaborwa massifs [Landa et al.,
1983; Dawson, Hinton, 2003]. Moreover, simple mass-balance calculations
show that no more than 5-10 wt.% of apatite/dolomite addition to
baddeleyite composition would result in observed REE distribution
(dashed lines on Fig.1b). One more argument in favor of such
interpretation of the data are the results of analysis of bulk
baddeleyite sample (100mg, ISP-MS from the solution) represented on the
same diagram (Fig.1b, open circles).
At the same time trace
element distributions for the studied carbonatite and foscorite-hosted
baddeleyite do not demonstrate any significant HREE enrichment ((Lu/La)n
= 23.8, (Lu/Gd)n= 5.4) that is typical for baddeleyite of
definite metamorphic origin [Rubatto, Scambelluri, 2003]. But ∑REE
varies considerably – from 7.8 to 160 ppm for Kovdor baddeleyite and
[Nb] from 980 to 2660, [Y] from 19.6 to 41.1, [Ti] from 17.6 to 47.9,
[Hf] from 7440 to 12160, [Th] from 1.96 to 13.7, [U] from 121 to 774
ppm, but [Sr] from 1.34 to 1.89 and [Ba] from 0.23 to 0.36 ppm which is
quite unlike to those for Phalaborwa baddeleyite.
Moreover, there is
surprising difference in the shape of a Ce positive anomaly for
baddeleyite and zircon REE patterns (Fig. 1). Zircon LREE normalized
distribution generally demonstrates the existence of a La-Ce-Pr peak.
The shape of this peak is determined by relative value of
chondrite-normalized La, Ce, Pr and Nd: Lan£Prn<Ndn<<Cen.
By comparison, baddeleyite REE patterns are characterized by La-Ce-Nd
anomaly also that is determined by relatively reduced Nd content (Lan£Ndn<Prn<<Cen).
But in-situ baddeleyite composition data are controversial even
for Phalaborwa baddeleyite standards. Rubatto and Scambelluri (2003)
reported the first type of Ce positive anomaly (zircon type); while Xie
et al. (2008) obtained both LREE distributions for Phalaborwa
baddeleyites. Trace element compositions for baddeleyite from other than
carbonatite rocks show analogous complex behavior [Rubatto, Scambelluri,
2003]. We produced Phalaborwa baddeleyite REE patterns that have only
zircon-type positive Ce anomaly (Fig. 1a) but Kovdor baddeleyite data
confirm also the presence of both La-Ce-Pr and La-Ce-Nd type of Ce
positive anomaly (Fig. 1b). The significance and reason for such Nd
behavior in baddeleyite crystal structure is not clear because
experimental data is not sufficient for this [Klemme, Mayer, 2003] but a
possible explanation could be connected with the magma’s middle REE
exhaustion during crystallization of such REE-rich minerals as
F-apatite, REE-pyrochlore, calzirtite, zirconolite and others. To prove
this hypothesis it is necessary to carry out further investigations of,
in particular, the character of baddeleyite composition evolution
accompanying the development in time of magmatic and ore-formation
processes.
The present study of
geochemical features of individual baddeleyite crystals from phoscorites
and carbonatites of the Kovdor massif (Fig.1) has demonstrated the
excess heterogeneity of their composition in comparison to those
variation for Phalaborwa massif (South Africa) baddeleyite which is
determined not only by the presence of microinclusions of carbonates and
apatite in the samples studied but, perhaps, by the difference in the
grains studied origin. But this could not exclude the possibility that
baddeleyite crystals formed within the rocks and ores of one magmatic
stage and representing only one morphological type are characterized by
narrow composition variations and have the single concordant U-Th-Pb
isotope age, i.e. they posses all the necessary features for
isotope-geochemical and geochronological standard.
References:
Arzamastsev A.A., Arzamastseva L.V., Bea F., Montero
P. Trace elements in minerals as indicators of the evolution of alkaline
ultrabasic Dike Series: LA-ICP-MS Data for the magmatic provinces of
Northeastern Fennoscandia and Germany. Petrology. 2009. V.17(1). P.
46–72.
Dudkin O.B., Kirnarsky
Yu.M. Kovdor Massif Deposits. Geology of Ore Deposits. 1994. V.36. №1.
P. 31-41.
Dawson J.B., Hinton R.W.
Trace-element content and partitioning in calcite, dolomite and apatite
in carbonatite, Phalaborwa, South Africa. Mineralogical Magazine. 2003.
V.67(5). P. 921–930.
Heaman L.M. The application
of U–Pb geochronology to mafic, ultramafic and alkaline rocks: an
evaluation of three mineral standards. Chemical Geology. 2009. V.261. P.
43–52.
Hoskin P.W.O.,
Ireland T.R. Rare earth element chemistry of zircon and its use as a
provenance indicator. Geology. 2000. V. 28. No.7. P. 627-630.
Kempe U., Götze J.
Cathodoluminescence (CL)
behaviour and crystal chemistry of apatite from rare-metal deposits.
Mineralogical Magazine.
2002. V.66. P. 151–172.
Kirnarsky Yu.M. Some
peculiarities of distribution and composition of accessory baddeleyite
from carbonatites. In: New data about Kola Peninsula minerals. Apatity.
1979. P. 76-82 (in Russian).
Klemme S., Meyer H.-P.
Trace element partitioning between baddeleyite and carbonatite melt at
high pressures and high temperatures. Chemical Geology. 2003. V.199. P.
233–242.
Krasnova N.I., Kopylova
L.N. The geological basement for mineralogical mapping survey (Kovdor
deposit). Reports of Academy of Sciences of USSR. Geology. 1988. №5. P.
81-92.
Kopylova L.N., Krasnova
N.I., Martovitskaya N.A., Poritskaya L.G. Typical chemical features of
calcite and baddeleyite of the Kovdor complex deposit. In: «Alkaline
magmatism and apatite potential of the North of Siberia». Proceedings of
NIIGA. L-d. 1980. P. 124-138 (in Russian).
Landa E.A., Krasnova N.I.,
Tarnovskaya A.N., Shergina Yu.P. REE and Y distribution in apatite from
alkaline-ultramafic massifs and carbonatites and genesis of apatite
mineralization. Geochemistry. 1983. №1. P. 91-101.
Reischmann T., Brugmann
G.E., Jochum K.P., Todt W.A. Trace element and isotopic composition of
baddeleyite. Mineral Petrology. 1995. V.53, P. 155–164.
Rimskaya-Korsakova O.M.,
Dinaburg I.B. Baddeleyite in ultrabasic and alkaline massifs of the Kola
Peninsula. In: Mineralogy and geochemistry. Issue 1. L-d. 1964. P. 13-30
(in Russian).
Rodionov N., Belyatsky B.;
Antonov A., Kapitonov I., Sergeev S. Comparative in-situ U-Th-Pb
geochronology and trace element composition on baddeleyite and low-U
zircon from the carbonatite Paleozoic Kovdor Massif (Kola Peninsula).
Gondwana Research. 2012. V.21. P. 728-744.
Rubatto D., Scambelluri M.
U-Pb dating of magmatic zircon and metamorphic baddeleyite in the
Ligurian eclogites (Voltri Massif, Western Alps). Contributions to
Mineralogy and Petrology. 2003. V.146. P. 341–355.
Xie L.W., Zhang Y.B., Zhang
H.H., Sun J.F., Wu F.Y. In situ simultaneous determination of trace
elements, U-Pb and Lu-Hf isotopes in zircon and baddeleyite. Chinese
Science Bulletin. 2008. V.53. P. 1565–1573. |